Research
Energy sustainability is a vital challenge for humanity. Solving this question requires materials able to convert and store energy efficiently. We develop computer models to expedite the deployment of materials for energy technologies, working at the interface between materials science, physics, and chemistry in close collaboration with experimentalists.
Large-Scale Simulation
of Layered Photocatalysts
Solar energy is the most abundant energy source available to humankind, but this energy cannot be harnessed on demand due to the variability of sunlight. Artificial photosynthesis overcomes that variability through the direct photocatalytic storage of solar power into chemical fuels; however, most of the stable photocatalysts in use today rely on metal oxide semiconductors whose bandgap does not match the solar spectrum. This research is focused on understanding and improving the performance of layered oxides that can operate optimally under sunlight.
Sponsor | NSF (CAREER);
Principal Investigator | Ismaila Dabo.
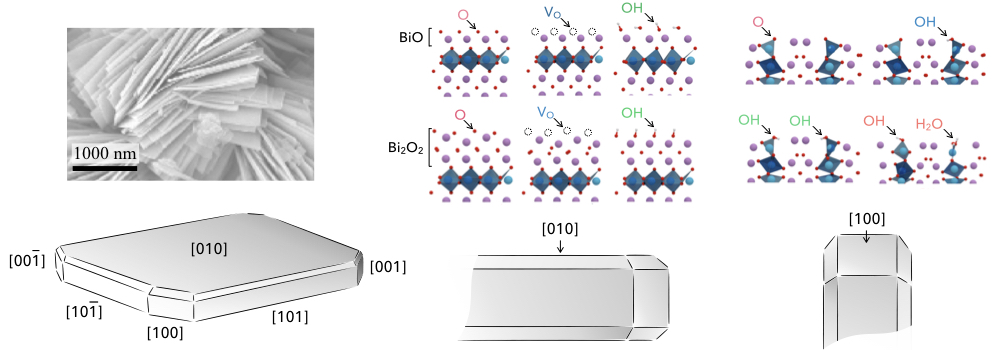
Figure | Layered bismuth oxides are efficient light absorbers for producing hydrogen using solar energy. Experimental microscopy (Saison et al., 2013) reveals that these oxides adopt a flake-like morphology (top left). We have developed a predictive computer model to assess the ability of these nanoparticles to separate the photogenerated charges as a function of the structure of the exposed facets (bottom left, and right).
Voltage-Dependent Stability
of Electrocatalysts
The electrochemical oxidation of transition-metal electrodes is a major concern for the durability of fuel cells and a crucial step in the operation of metal–air batteries. Growing out the compelling need to describe the durability of transition metals in aqueous media, the goal of this research is to exploit and further develop quantum–continuum models and voltage-dependent reactive force fields to elucidate the electrochemical stability and dynamical restructuring of noble metals under applied voltage. This work is in collaboration with the Sinnott group.
Sponsor | DOE (CPIMS);
Principal Investigator | Ismaila Dabo.
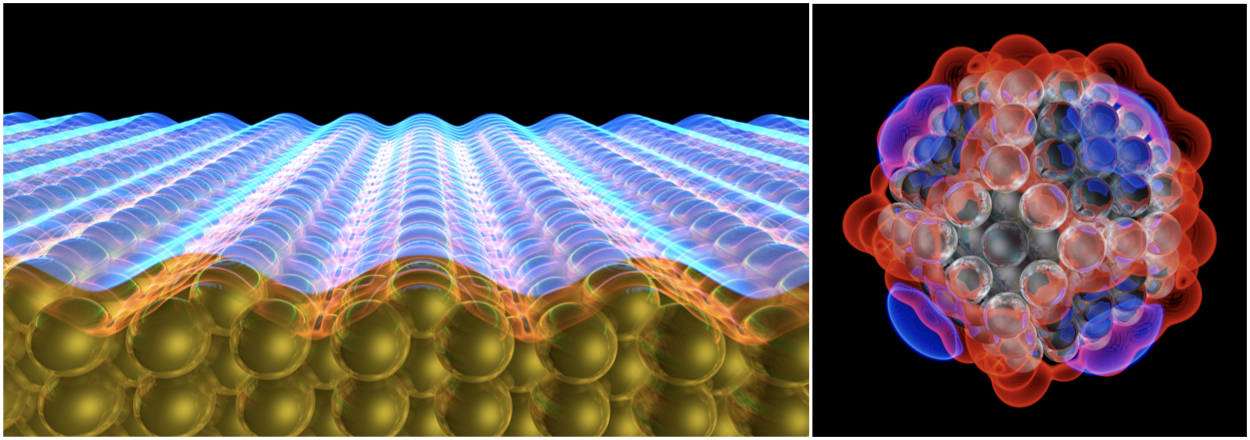
Figure | The stability of catalytic electrodes is critical to the performance of electrochemical energy technologies. A quantum–continuum model of the solid–liquid interface has been applied to effectively represent the polarization of a reconstructed Au(110) electrode under applied voltage (left). The quantum-continuum model has been used to describe the potential surrounding a truncated octahedral Pt particle (right).
High-Throughput Search
for Water Splitting Materials
The production of hydrogen fuel from sunlight and seawater has the potential to profoundly reshape the food–energy–water landscape and to revolutionize the generation of electric power for transportation and residential applications. Hydrogen is a sustainable energy carrier whose catalytic reaction with oxygen generates electricity and heat without emitting carbon dioxide; the only byproduct of this reaction is clean water. The goal of this research is to develop a high-throughput computational infrastructure to accelerate the selection of materials that can split water, in collaboration with the Abruña, Fennie, Gopalan, and Schaak groups.
Sponsor | NSF (DMREF);
Principal Investigator | Ismaila Dabo.
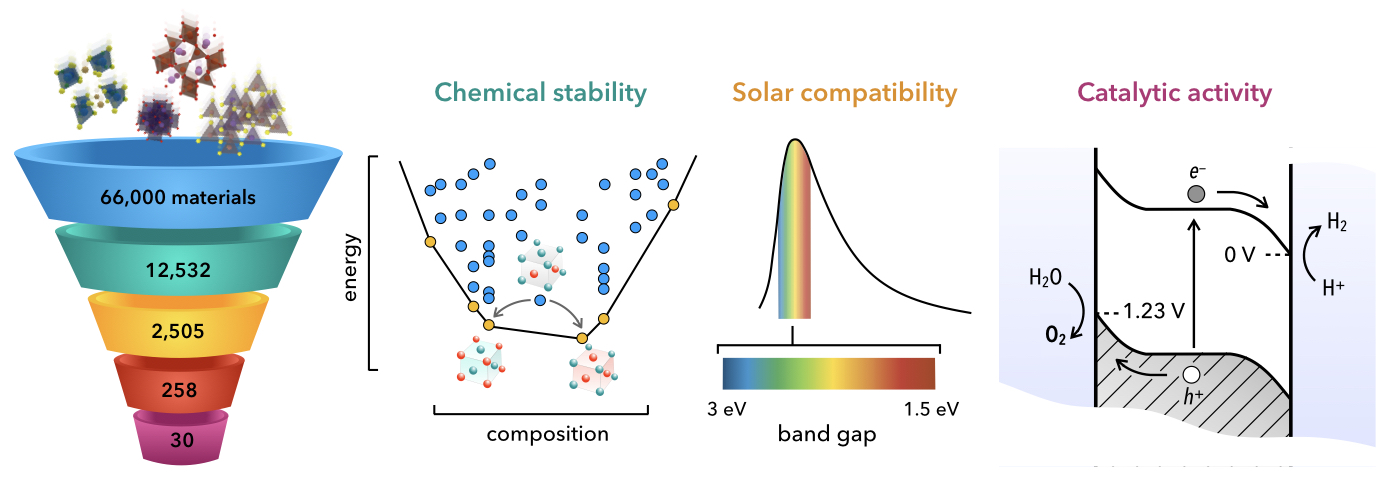
Figure | Computational screening of semiconductor photoelectrodes for producing renewable hydrogen. Starting with 66,000 materials from the Materials Project database, 30 candidate photocatalysts have been screened (left) by computing the chemical stability, solar compatibility, and catalytic activity of the candidate semiconductor electrodes (right).
First-Principles Prediction
of Pseudocapacitive Energy Storage
The development of advanced energy storage technologies combining the energy density of batteries with the power density of supercapacitors is critical to overcome the frontier separating the performance of electrochemical systems from that of internal combustion engines and to meet the technical requirements for electric transportation and grid energy storage. Pseudocapacitors are electrochemical devices characterized by fast and reversible redox reactions that enable them to store large amounts of electrical energy at high rates. This industry-sponsored project aims to predict the microscopic mechanisms of energy storage in pseudocapacitors.
Sponsor | Murata Manufacturing Co., Ltd.;
Principal Investigator | Ismaila Dabo.
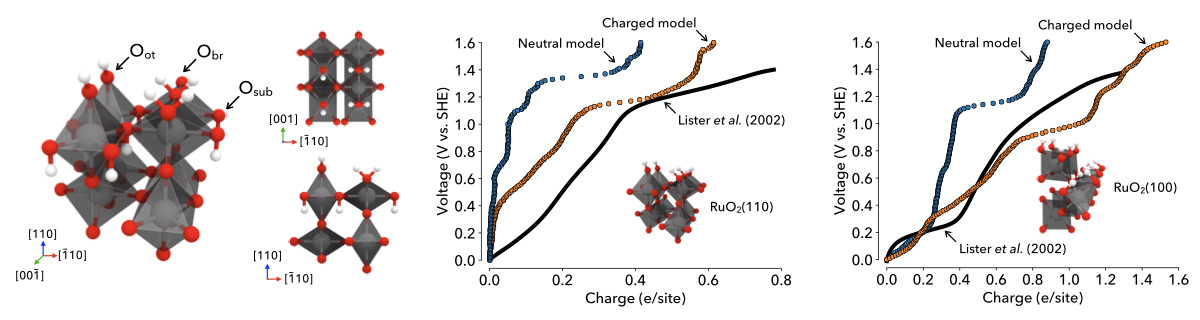
Figure | The energy density of ruthenia pseudocapacitor electrodes depends on their surface structure. To predict this dependence, we have computed the electrochemical response of ruthenia surfaces in aqueous environments. Simulations using neutral-interface (blue) and charged-interface (orange) models are compared to experimental results, illustrating the accuracy of charged-interface calculations.